Open Access | Review
This work is licensed under a Creative
Commons Attribution-ShareAlike 4.0 International License.
Advances in targeting programmed cell death 1/programmed cell death-ligand 1 therapy for hematological malignancies
# The first two authors contributed equally to this work.
* Corresponding author: Liang Wang
Mailing address: Department of Hematology, Beijing Tongren
Hospital, Capital Medical University, Beijing 100730, China;
Beijing Advanced Innovation Center for Big Data-Based Precision
Medicine, Beihang University & Capital Medical University,
Beijing Tongren Hospital, Beijing 100730, China.
Email: wangliangtrhos@126.com
Received: 15 November 2021 / Accepted: 06 December 2021
DOI: 10.31491/APT.2021.12.071
Abstract
Programmed cell death 1 (PD-1) and programmed cell death-ligand 1 (PD-L1) are important immune checkpoints, and their interactions can mediate immune suppression in the tumor microenvironment. Targeting PD-1 and PD-L1 are immune checkpoint inhibitors that bind to PD-1 and PD-L1, respectively, to block the signal pathway between the two and increase the immune response. They are widely used in tumor treatment and have good efficacies for malignant melanoma, renal cell carcinoma, and non-small cell lung cancer, among others. In addition, for hematological malignancies, studies targeting PD-1 and PD-L1 have achieved gratifying results. This article briefly reviews the mechanisms of action and clinical and hematological malignancy applications of targeting PD-1 and PD-L1.
Keywords
PD-1, PD-L1, mechanism of action, hematological malignancy
Introduction
In recent years, tumor immunotherapy has gradually become a hot spot in the field of tumor treatment and has shown good prospects in cancer treatment [1], which is also one of the most promising research directions in tumor treatment. Programmed cell death 1 (PD-1; also known as CD279 [2]) is a member of the B7 receptor family. Its ligands are programmed cell death-ligand 1 (PD-L1; also known as CD274) and programmed cell death-ligand 2 (PD-L2; also known as CD273), which are expressed by multiple types of cells [3]. Among them, PD-1 and PD-L1 are the most common immune checkpoints, which mainly inhibit stimulation, negatively regulate T-cell functions, Aging Pathobiology and Therapeutics 2021; 3(4):84-94 84 DOI: 10.31491/APT.2021.12.071 and weaken the immune response to tumors. Therefore, targeting PD-1 and PD-L1 can block the combination of PD-1 and PD-L1 to restore the antitumor effect of the immune system. This article briefly reviews its mechanism of action and application in hematological malignancies.
Mechanism and overview of PD-1/PD-L1
Structural features
PD-1 is a transmembrane protein with 288 amino acids
and belongs to the immunoglobulin superfamily. It is
present in lymphocytes, natural killer (NK) cells, monocytes,
and dendritic cells [4]. PD-1 contains an Ig variable-
type extracellular domain, a transmembrane domain,
and a cytoplasmic tail [5]. The cytoplasmic tail has an
immunoreceptor
tyrosine-based inhibitory motif (ITIM) [6]
and immunoreceptor tyrosine-based switch motif (ITSM).
After PD-1 binds to PD-L1, the ITIM and ITSM of PD-1
are phosphorylated by Src family tyrosine kinases [7],
thereby inhibiting T-cell activity and proliferation. Thus,
PD-1 has dual roles in immunological tolerance: induction
and maintenance of peripheral tolerance [8]. PD-1
can also bind to B7-H1 and the crystallizable fragment (Fc)
fusion protein to inhibit the production of interleukin-2
(IL-2) in lipopolysaccharide (LPS-stimulated RAW264.7 cells by inhibiting the Janus N-terminal kinase
signaling
pathway [9].
Programmed cell death-ligands (PD-Ls) contain PD-L1
and PD-L2. Whereas PD-L1 is constitutively expressed
and upregulated in T cells, B cells, macrophages, dendritic
cells, endothelial cells, epithelial cells, and muscle cells,
PD-L2 is only expressed on the surface of dendritic cells,
macrophages, and bone marrow-derived mast cells [10].
Moreover, they have similar exon organizations of the
5'-untranslated region; a signal sequence; IgV-like, IgClike,
and transmembrane domains; and cytoplasmic exons
1 and 2 with the 3'-untranslated region [11]. PD-L1 has
many functions; when it binds to PD-1, it can inhibit Tcell
proliferation and cytokine production [11]. PD-L1 can
also interact with B7-1. Regarding its mechanism of action,
many theories have been proposed, with some studies
suggesting that cis-PD-L1/B7-1 on antigen-presenting
cells (APCs) disrupts the transbinding of PD-1/PD-L1.
Through this mechanism, APCs expressing substantial
amounts of B7-1 mediate diminished T-cell inhibition via
PD-1 [12], which provides a relevant basis for combination
therapy with B7-1 and PD-L1. The role of the combination
of PD-L2 and PD-1 in regulating the differentiation
and function of T cells remains to be determined because
both coinhibitory and costimulatory functions have been
reported [13]. Therefore, drug designs are mainly focused
on PD-1 and PD-L1 targets. PD-L2 can also interact with
repulsive guidance molecule b (RGMb) to regulate respiratory
tolerance [13].
Mechanisms
PD-1 can bind to PD-Ls. When binding to a PD-1 ligand
on antigen-presenting cells, PD-1 can shut down self-reactive
T cells and induce peripheral tolerance. While binding
to a PD-1 ligand on parenchymal cells, PD-1 can maintain
tolerance and prevent tissue destruction by inhibiting effector
T cells [8]. The mechanisms of action of targeting
PD-1/PD-L1 are shown in Figure 1.
The PD-1 pathway has
various mechanisms of action.
After the ITIM and ITSM of PD-1 are combined, they are
phosphorylated by Src family tyrosine kinases, and Src
homology 2 domain-containing phosphatases (SHPs) are
further recruited to the phosphorylated tyrosine residue.
SHPs can dephosphorylate downstream signaling pathways,
thereby blocking cell cycle progression [14]. SHPs
can also inactivate zeta-chain-associated protein kinase 70
and protein kinase C-θ [2]. In addition, Francisco and colleagues
demonstrated that PD-L1 can induce and maintain
Tregs and enhance immune suppression at the organismal
level [15].
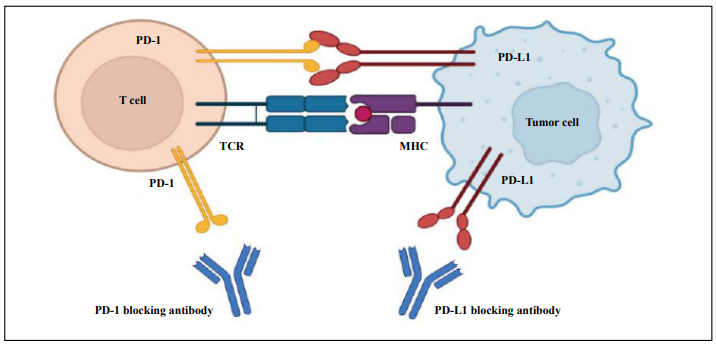
Figure 1. The mechanisms of action of targeting PD-1/PD-L1.
Clinical application
Immune checkpoint inhibitors have become a key method
of cancer treatment, especially targeting PD-1/PD-L1.
In recent decades, an increasing number of related drugs
have been used to treat malignant tumors.
Among PD-1 inhibitors, pembrolizumab [16] was first
approved by the Food and Drug Administration (FDA) in
September 2014 for the treatment of melanoma, non-small
cell lung cancer (NSCLC), and so forth. Three months
later, nivolumab was approved for release in the market
[16] for the treatment of melanomas that are unresponsive
to other drugs. In the same year, MPDL3280A was shown
to be a breakthrough therapy for metastatic bladder cancer
and NSCLC in February of the following year. In China,
Opdivo (nivolumab) was first approved for release in the
market in June 2018; and Keytruda (pembrolizumab), in
July. Thereafter, several drugs have been launched in the
market.
Among PD-L1 inhibitors, atezolizumab was first approved
by the FDA for the treatment of bladder cancer, followed
by durvalumab, avelumab, and so forth. At present, two
PD-L1 inhibitors have been approved for release in the
market in China, namely Imfinzi (durvalumab) in December 2019 and Bavencio (atezolizumab) in February
2020.
However, with the progress of drug research, drug resistance
has gradually become an urgent problem to be
solved. Research has shown that the tumor-immune cycle
is divided into the following seven steps: the release of
cancer antigens, cancer antigen presentation, priming and
activation, trafficking of T cells to tumors, infiltration
of T cells into tumors, recognition of cancer cells by T
cells, and killing of cancer cells. Given that PD-1/PD-L1
blockade is primarily involved in step 7, any abnormalities
in the previous steps may lead to drug resistance [17],
such as lack of tumor antigen expression, dysbiosis of
the normal gut microbiome, and migration disorders of T
cells. Therefore, for combination drugs, the PD-1/PD-L1
pathway can be used as the cornerstone of the combined
checkpoint blockade program to antagonize additional
inhibitory signals and thereby improve the immunity efficacy
of checkpoint blockade in cancer treatment [18]. At
present, the most commonly used combination drugs are
as follows: Radiotherapy can cause the release of tumor
antigens and increase the immunogenicity of tumors [19].
Vaccines, CD40 agonists, and toll-like-receptor agonists
can promote dendritic cell (DC) cross-presentation to enhance
the anti-tumor response of T cells. Cytotoxic T-lymphocyte
antigen-4 (CTLA-4) blockers, CD137 agonists,
and inflammatory cytokines such as IL-2 can enhance the
initiation and activation of T cells. Block inhibitory immune
checkpoint molecules such as T-cell immunoglobulin
and mucin domain 3 (TIM-3) and lymphocyte-activation
gene 3 (Lag-3) [17] can reduce the drug resistance of
targeting PD-1/PD-L1 and enhance the therapeutic effect.
Application of targeting PD-1/PD-L1 in hematological malignancies
With the progress of drug research, targeting PD-1/PDL1
has gradually been used in hematological malignancies.
Relevant clinical trials have been conducted for
drugs such as nivolumab and pembrolizumab, and some
drugs have been approved for use in the clinical treatment
of certain hematological malignancies. According to research,
for hematological malignancies, targeting PD-1/
PD-L1 is most effective for lymphoma, followed by leukemia,
but least effective for multiple myelomas (MMs).
This may be related to the expression levels of PD-L1 in
different types of tumors and in the tumor microenvironment.
Several studies have examined the usefulness
of PD-L1
expression levels. Immunohistochemistry (IHC) assays
have demonstrated that response rate directly correlates
with PD-L1 expression level. In addition, patients with
high PD-L1 expression levels in tumor-infiltrating immune
cells showed better therapeutic responses and clinical
outcomes [20]. However, the expression levels of PDL1
on neoplastic cells and in the tumor microenvironment
vary between different subtypes, and the prognostic implications
of PD-L1 expression remain unclear [21]. Future
studies need to further delineate the mechanisms of PD-L1 expression and explore more possibilities in depth.
In addition, the therapeutic effect of targeting PD-1/PDL1
may also be related to the tumor microenvironment,
in which inflammatory cytokines, especially interferon γ
(IFN-γ), are present. Inflammatory cytokines stimulate not
only tumor cells that express PD-L1 and PD-L2 but also
other types of cells in the tumor microenvironment, such
as macrophages, dendritic cells, and stromal cells, that increase
the expression level of PD-1 ligand [22].
Therefore, among
hematological malignancies, lymphoma
has the best therapeutic response. In particular, the copy
numbers of the PD-L1 and PD-L2 gene loci in most patients
with Hodgkin lymphoma (HL) are amplified, which
leads to the upregulation of PD-L1 and PD-L2 expressions.
Therefore, targeting PD-1/PD-L1 has a good therapeutic
effect on HL. In non-HL (NHL), targeting PD-1/
PD-L1 has a good therapeutic effect on diffuse large Bcell
lymphoma (DLBCL). Nearly 20% of DLBCLs not
otherwise specified (NOS) have PD-L1 overexpression,
and the structural abnormality of the 9p24.1 chromosome
is significantly related to PD-L1 expression. Targeting
PD-1/PD-L1 also has a good therapeutic effect on NK/Tcell
lymphoma (NKTCL). The mRNA levels of PD-L1
and PD-L2 in patients with NKTCL are significantly upregulated.
However, patients with mantle cell lymphoma
(MCL) have weaker PD-L1 and PD-L2 on the surfaces of
B and T cells than healthy individuals, and experiments
have shown that, targeting PD-1/PD-L1 is ineffective in
these patients. Targeting PD-1/PD-L1 has a weaker therapeutic
effect on leukemia than lymphoma. Patients with
acute myeloid leukemia (AML) have a significant increase
in PD-1 expression on T cells due to the imbalance of the
PD-1 pathway and the tumor microenvironment. Targeting
PD-1/PD-L1 has the weakest therapeutic effect on MMs.
Experiments have shown that in patients with MMs, the
expression levels of PD-L1 in plasma cells are different
and higher than those in healthy volunteers.
Regarding the specific application of targeting PD-1/PDL1
to hematological malignancies, this article describes its
effects.
Lymphoma
(1) Hodgkin lymphoma
For most HLs, cytogenetic studies have shown that the copy numbers of the gene loci of PD-L1 and PD-L2 are amplified, which leads to the upregulation of the surface expressions of PD-L1 and PD-L2 [23]. Therefore, targeting PD-1/PD-L1 has a better therapeutic effect on HLs.
1) Relapsed/refractory HL
Currently, nivolumab and pembrolizumab are approved
by the FDA for the treatment of advanced relapsed/refractory
(r/r HL). In addition, a previous study found that 70%
of patients with r/r HL responded to PD-1 inhibitors, of
whom 20% had a complete response [24].
A retrospective study
included 53 patients with r/r HL
from nine US centers who were treated with nivolumab.
The overall efficacy rate obtained by the discoverer was 68%; 12-month progression-free survival rate, 75%;
overall
survival rate, 89%; complete remission rate, 45%; and
partial remission rate, 23% [25], indicating that nivolumab
has a good treatment effect on HL. A clinical trial published
by the European Hematology Association (Abstract:
PF431) studied the use of nivolumab and brentuximab
vedotin (BV) as combination therapy for the treatment of
10 patients after failure of nivolumab monotherapy. The
median follow-up time was 10.8 months (range, 7.4-13
months). The objective response rate of the treated patients
was 70%, the complete response rate was 30%, and
nine patients (90%) had treatment-related adverse events
(AEs), of which nausea and peripheral neuropathy were
the most common. This shows that the therapeutic effect
of nivolumab + BV is similar to that of monotherapy.
Thus, nivolumab + BV is expected to be an effective
rescue plan for patients with r/r HL who have failed to
respond to nivolumab monotherapy. In addition, a recent
study found that for 59 patients with r/r HL treated with
nivolumab and BV after autologous hematopoietic cell
transplantation, the 18-month progression-free and overall
survival rates were 95% and 98% [26], respectively, indicating
that nivolumab is also expected to be used for the
consolidation of autologous hematopoietic cell transplantation.
Pembrolizumab therapy is also effective.
A multi-cohort
phase II study found that pembrolizumab has clinical activity
in patients with r/r HL, with an objective response
rate of 65-72% and a complete response rate of 22%
[27]. A phase I clinical study found that after treatment
with pembrolizumab, of 31 patients who failed treatment
with an anti-CD30 monoclonal antibody, 90% had tumor
shrinkage, with a total efficacy rate of 65% [28], showing
a satisfactory result. Pembrolizumab can also be combined
with other drugs. A phase II study found that 32 (94%) of
34 patients achieved complete remission after using pembrolizumab-
GVD (gemcitabine, vinorelbine, and liposomal
doxorubicin) as a second-line treatment for r/r HL [29].
This shows that the treatment is better than the standalone
treatment and is an efficient and well-tolerated program.
In addition to the two above-mentioned drugs,
many drugs
are effective for the treatment of r/r HL. A study used
sintilimab to treat 96 patients with r/r cHL and showed
that as of September 30, 2019, 57.3% of patients completed
the treatment. After 2 years of treatment, the 2-year
overall survival rate was 96.3%. The long-term follow-up
results showed that in addition to the high response rate,
sintilimab also showed long-lasting efficacy and good
long-term safety [30]. A clinical study used camrelizumab
combined with decitabine to treat 51 patients with r/r cHL
who failed treatment with anti-PD-1 therapy. The median
progression-free survival time with the combination
therapy was significantly longer than that with the previous
anti-PD-1 monotherapy. In patients who achieved
complete remission at 24 months, 78% observed a durable
response [31], indicating that in patients with r/r cHL, the
use of camrelizumab and decitabine as third-line therapy
or beyond has tolerable and significant antitumor activity.
2) Untreated HL
With the widespread application of targeting PD-1/PDL1 in r/r HL, an increasing number of clinical trials have investigated its therapeutic effect on newly treated HL. In a recent phase II study, nivolumab and BV combination therapy in patients older than 60 years among the evaluable patients, the best objective response rate was 95%, proving that BV-nivo is effective for elderly patients with untreated HL who have comorbidities [32].
(2) Non-Hodgkin lymphoma
NHL is divided into B-, T-, and NK/T-cell lymphomas. B-cell lymphomas include DLBCL, follicular lymphoma (FL), and MCL. This article elaborates on these.
1) Diffuse large B-cell lymphoma
In DLBCLs, PD-L1 can be expressed by B cells in DLBCL
tumors and non-malignant cells (e.g., macrophages)
from its immune microenvironment [33]. According to
reports, nearly 20% of DLBCL NOS have genetic abnormalities
and chromosomal changes, which lead to PDL1
overexpression [34]. Among these abnormalities, the
structural abnormality of the 9p24.1 chromosome is significantly
related to the expression of PD-L1 in DLBCL
[35].
For the treatment of DLBCL, preliminary trials have
shown that PD-1 inhibitors have limited effects on
DLBCL. Nivolumab can be used alone, as a phase I study
found that the use of nivolumab alone in the treatment
of 81 patients with lymphoma and myeloma (11 cases of
DLBCL) had an efficacy rate of 36% [36]. Nivolumab can
also be used in combination therapies. In the combined
use of nivolumab and ipilimumab in the treatment of 65
cases of hematological malignancies (10 cases of DLBCL),
only three cases responded [37], indicating a poor
treatment effect. Recently, a study (NCT03305445) that
included six patients with DLBCL treated with nivolumab
and ipilimumab after conventional treatment and before
stem cell transplantation showed that three (50%) of the
patients benefited from and well tolerated the transplantation,
indicating an acceptable effect. A clinical trial evaluated
pembrolizumab combined with rituximab plus cyclophosphamide,
doxorubicin, vincristine, and prednisone
(R-CHOP) in the treatment of 30 patients with untreated
DLBCL and revealed that the therapy had comparable toxicity
with the standard R-CHOP therapy, but two patients
developed grade 3 immune-related AEs. The overall and
complete response rates were 90% and 77%, respectively,
and the 2-year progression-free survival rate was 83%
[38], which was better than pembrolizumab monotherapy.
Several later studies were conducted to examine the effect
of pembrolizumab combined with other drug treatments.
In addition to the two abovementioned drugs, many
other
drugs have been studied for the treatment of DLBCL. A
study of zanubrutinib in combination with tislelizumab in
69 patients with B-cell malignancies (including 27 with
DLBCL) showed an objective remission rate of 37% in
patients with DLBCL, of whom four (14.8%) had a complete remission and six (22.2%) had a partial remission
[39]. In germinal center B-cell-like (GCB) and non-GCB
(NGCB) DLBCLs, the objective remission rates were
33.3% and 40%, respectively, indicating a poor therapeutic
effect. A clinical trial of atezolizumab combined with
R-CHOP in the treatment of 42 patients with untreated
DLBCL showed that 31 patients (77.5%) achieved complete
remission and four (10%) achieved partial remission
according to an independent review committee [40],
which indicate a promising effect.
In some special types of DLBCL, targeting PD-1/PD-L1
has a better curative effect. In primary central nervous
system lymphoma (PCNSL), owing to the changes in
chromosome 9p24.1 [41], the PD-L1 expression level is
increased, so targeted therapy drugs have a better effect.
A clinical trial that used nivolumab to treat four patients
with r/r PCNSL and one patient with relapsed primary testicular
lymphoma (PTL) revealed that all five patients had
objective responses, including complete remission in four
patients and partial remission in one patient [42], indicating
that the treatment has high efficacy, had a long-lasting
curative effect, and can improve central symptoms at the
same time. However, a phase II trial of nivolumab for r/r
PCNSL or r/r PTL showed that the objective response rate
assessed by blinded independent central review (BICR)
was 6.4%, and the progression-free survival period was
1.41 months [43], indicating that the efficacy of targeting
PD1/PD-L1 in PCNSL must be confirmed by more
prospective clinical studies. An ongoing phase II clinical
trial of nivolumab and ibrutinib for r/r PCNSL is currently
recruiting patients [44].
Primary mediastinal large B-cell
lymphoma (PMBCL) is
similar to PCNSL because of the chromosome changes
that lead to the overexpression of PD-L1; thus, the application
of targeted drugs has a better effect. According
to a report, the objective response rate of the first batch
of 19 patients in the PMBCL cohort was 41% [45]. The
National Comprehensive Cancer Network guidelines recommend
pembrolizumab for r/r PMBL. A phase II trial
of pembrolizumab in the treatment of 53 patients with r/
r PMBL showed an objective response rate of 45% and a
complete response rate of 21% [46], indicating effective
outcomes. A clinical trial of nivolumab combined with BV
in the treatment of 30 patients with r/r PMBL showed that
at a median follow-up of 11.1 months, the objective response
rate was 73%, the complete response rate assessed
by each investigator was 37%, and the remission rate was
70% [47], which is better than the treatment alone.
The
overexpression of PD-L1 in Epstein-Barr virus
(EBV)-associated lymphoma [48] is thought to be mediated
by the latent membrane protein 1 (LMP1) encoded
by EBV [49], which makes it sensitive to PD-1 blockade.
An experiment was conducted to study the effect of PD-1
blockade on the antitumor immunity of lymphoma cells
and revealed that PD-1 blockade exerted a highly effective
role in EBV+ DLBCL [50], stronger than that of EBV-DLBCL, indicating that targeting PD-1/PD-L1 will have
a better effect on DLBCL. We look forward to conducting more clinical trials to verify the efficacy of the
drug in the
future.
2) Follicular lymphoma
Unlike DLBCL, most FL tumor cells do not express PDL1
or PD-L2 [33], but the immune infiltrates expressed
PD-L1 and PD-L2 at normal levels and overexpressed
PD-1 [33]. Cells expressing PD-1 include not only cells
derived from tumor-infiltrating lymphocytes (TILs) but
also follicular helper T cells from lymphoma follicles or
residual germinal centers [51]. In addition, PD-1+ TILs in
FL and DLBCL positively correlate with prognosis, and
the presence of PD-1+ TILs in lymphoid tumors may indicate
the source of the cells [52].
Regarding the treatment of FL, a
phase I study of
nivolumab for the treatment of r/r hematological malignancies
(including 10 patients with recurrent FL) showed
an objective remission rate of 40% [36]. A phase II clinical
trial found that the use of nivolumab as the first-line
treatment for immune initiation, followed by treatment
with nivolumab and rituximab, in 39 patients with FL
at a median follow-up time of 17.5 months achieved an
overall response rate of 92%, a remission rate of 54%, and
a median remission time of 5 months. In 25 evaluable patients,
the 12-month progression-free and overall survival
rates were 72% and 96% [53], respectively, indicating that
the effect of the regimen is better than that of nivolumab
alone. A phase II clinical trial demonstrated that pidilizumab
combined with rituximab in the treatment of 30
patients with relapsed FL had a total efficacy rate of 66%,
with 52% of the patients achieving complete remission
and 14% achieving partial remission [54], indicating a
good overall effect.
3) Mantle cell lymphoma
On the basis of experiment results, PD-L1 and PD-L2 expressions on the surface of B and T cells in patients with MCL are weaker than those in healthy individuals [55]. Therefore, these results show that targeting PD-1/PD-L1 is not effective for the treatment of MCL and should not be used as a related drug target.
4) T-cell lymphoma
Studies have shown that TCL overexpresses PD-1 [56] according
to the severity of the disease, so targeting PD-1/
PD-L1 has a certain therapeutic effect on TCL. Targeting
PD-1/PD-L1 is mostly used for r/r peripheral T-cell lymphoma
(PTCL). A phase I clinical study used nivolumab
to treat 23 patients with r/r TCL (of whom five had PTCL)
and showed that the partial response rate of the patients
with PTCL was 40% [36]. A clinical trial (NCT03075553)
used nivolumab in the treatment of 12 patients with r/r
PTCL and showed that the response rate of participants
who achieved complete or partial remission was 33.3%,
proving that nivolumab is effective for the treatment of
PTCL. Pembrolizumab combined with romidepsin has
been approved by the FDA for the treatment of r/r PTCL.
It has been applied in the treatment of r/r PTCL in a phase I/II clinical study (NCT03278782) with 15
evaluable patients,
with an objective response rate of 44% and a complete
remission rate of 20%.
Regarding untreated T-cell lymphoma, a previous study
showed a significant increase in PD-1 expression in peripheral
CD4+ and CD8+ T cells. After treatment, the
decline in PD-1 expression level was similar to that in
healthy people, so we can infer that targeting PD-1/PD-L1
also has a good effect on T-cell lymphoma. Few clinical
trials have been conducted on the treatment of untreated
T-cell lymphoma, and targeting PD-1/PD-L1 is expected
to have more applications in the future.
5) NK/T cell lymphoma
The mRNA expression levels of PD-L1 and PD-L2 in
extranodal NKTCL (ENKTCL) were significantly upregulated,
and studies have found that PD-L1 protein is
expressed in tumor cells in patients with ENKTCL [57].
Moreover, the expression level of soluble PD-L1 after
treatment is a useful biomarker for monitoring patients
with minimal residual disease [58].
Targeting PD-1/PD-L1 for
the treatment of r/r ENKTCL
has a good effect. Nivolumab therapy has been proven to
be effective for ENKTCL [59]. A clinical trial of nivolumab
in the treatment of three cases of r/r ENKTCL demonstrated
a complete response in two patients and a partial
response in one patient. Targeting PD-1 has also been
proven to be effective for r/r ENKTCL for which treatment
with L-asparaginase had failed [60]. In a retrospective
case report, seven patients with r/r NKTCL achieved
an objective response rate of 100% after seven treatment
cycles [60]. The therapeutic effects of other drugs subjected
to clinical trials were not as good as those of nivolumab
and pembrolizumab. In a phase II trial, avelumab achieved
a complete remission rate of 24% and an overall response
rate of 38% in the treatment of 21 patients with r/r ENKTCL
[61]. A clinical trial (NCT03228836) of IBI308 in
the treatment of 28 patients with r/r ENKTCL revealed a
progression-free survival period of 30 months.
A study showed that the use of targeting PD-1 and
pegaspargase, gemcitabine, and oxaliplatin (P-GEMOX)
as a combination therapy for the treatment of nine patients
with advanced ENKTCL achieved significant remission in
eight patients, including complete remission in seven and
partial remission in one [62]. A clinical trial of sintilimab
combined with chidamide in the treatment of 41 patients
with r/r ENKTCL revealed an objective response rate of
58.3%, a complete response rate of 44.4%, and a partial
response rate of 13.9% [63]. These results show that sintilimab
also has a beneficial effect in combination therapies.
Leukemia
(1) Acute myeloid leukemia
Most patients with AML can achieve complete remission
after conventional chemotherapy, and allogeneic hematopoietic
stem cell transplantation is the only treatment
option for AML [64]. In recent years, the development of targeting
PD-1/PD-L1 has also made significant
achievements
in the treatment of AML.
Studies have shown that the PD-1 pathway is abnormally
expressed in AML. Mouse leukemia cell C1498 expresses
low levels of PD-L1 when cultured in vitro but expresses
elevated PD-L1 levels when cultured in vivo, which suggests
that the PD-L1 expressions in leukemia cells benefit
from the tumor microenvironment [65]. Clinical data also
support the dysregulation of the PD-1 pathway in AML.
Compared with healthy people, patients with AML have
significantly higher PD-1 expression levels on T cells [66].
In addition to the PD-1 pathway, CTLA-4 and TIM-3 [2]
are also involved in the pathogenesis of AML.
Clinical trials are ongoing to determine the effectiveness
of targeting PD-1/PD-L1 for the treatment of AML, and
its individual and combination treatments have shown
positive results. A clinical trial showed that the use of
nivolumab monotherapy as maintenance treatment in
14 patients with AML (not eligible for transplantation)
achieved 6- and 12-month complete remission rates of
79% and 71%, respectively [67]. A phase II clinical study
showed that the objective remission rate of nivolumab
combined with azacytidine in the treatment of r/r AML
was 33% [68]. A clinical trial (NCT02464657) of idarubicin
and cytarabine with nivolumab for the treatment of
myelodysplastic syndrome and AML showed a relapsefree
survival time of 18.54 months (range, 8.20-23.22
months) and an overall survival time of 18.54 months
(range, 10.81-28.81 months).
A clinical trial (NCT02708641) of pembrolizumab in the
treatment of 12 patients with AML showed a relapse time
of 12.14 months. A clinical trial (NCT02996474) of pembrolizumab
combined with decitabine in the treatment of
10 patients with r/r AML demonstrated that the regimen
was feasible for all 10 people, indicating that the combined
drug effect was better.
(2) Acute lymphocytic leukemia
Little is known about the role of targeting PD-1/PD-L1 in acute lymphocytic leukemia (ALL), and only a few relevant clinical trials have been conducted. Currently, a clinical trial (NCT02879695) of nivolumab and blinatumomab or nivolumab and ipilimumab in the treatment of patients with B-cell acute lymphoblastic leukemia is recruiting participants. A clinical trial (NCT04546399) of nivolumab combined with blinatumomab for the treatment of relapsed B-cell acute lymphoblastic leukemia is also recruiting participants. A phase I trial (NCT02819804) of nivolumab combined with dasatinib in the treatment of patients with ALL [69] was terminated because its efficacy could not be evaluated and analyzed. Another clinical trial (NCT03160079) of pembrolizumab combined with blinatumomab for the treatment of adult r/r B-cell acute lymphoblastic leukemia is also currently recruiting participants, and more related trials can be expected in the future.
(3) Chronic myeloid leukemia
Studies have shown that in chronic myeloid leukemia
(CML), PD-L1 expression is upregulated in bone marrow
cells, and PD-1 is present on T cells [70]. However, little
is known about the effectiveness of targeting PD-1/PD-L1
for CML. Some scholars have shown that the use of antigen-
pulsed autologous dendritic cells or direct application
of in vitro transcribed RNA encoding leukemia-related
antigens combined with targeting PD-1 may help obtain a
stronger immune response and better clinical results [71].
A
clinical trial (NCT02011945) of nivolumab
combined
with dasatinib in the treatment of 16 patients with CML
showed no incidence of dose-limiting toxicity. A clinical
trial (NCT03516279) of pembrolizumab, dasatinib, and
imatinib mesylate or nilotinib for the treatment of CML is
currently conducting recruitment, and more related trials
are expected in the future.
(4) Chronic lymphocytic leukemia
Ramsay [72] confirmed that the expression of PD-1 on
CD3+ cells in patients with chronic lymphocytic leukemia
(CLL) was significantly higher than that in healthy
individuals. PD-1 expression was found to be a feature of
CD4+ and CD8+ T-cell exhaustion in CLL, which inhibits
CD4+ and CD8+ T cells from producing certain cytokines
(IFN-γ and tumor necrosis factor [TNF]) [73].
However, the
efficacy rate of targeting PD-1/PD-L1 for
CLL is low. A phase II study (NCT02332980) reported
that pembrolizumab was ineffective for CLL but effective
for Richter's syndrome (RS) because patients with RS
have higher PD-L1 expression levels and low T-cell receptor
clonality [74]. Currently, two trials (NCT03153202 and
NCT03514017) of pembrolizumab combined with ibrutinib
in the treatment of patients with CLL are conducting
recruitment. However, no clinical trial (NCT04781855) of
the combination of ipilimumab, ibrutinib, and nivolumab
for the treatment of CLL has yet been conducted. A clinical
trial (NCT03884998) of copanlisib combined with
nivolumab in the treatment of patients with NHL in CLL
is currently recruiting. Research on immunotherapy for
patients with CLL is still ongoing, and more data to guide
treatment needs must be confirmed through further research
[75].
Multiple myeloma
Studies have shown that the expression level of PD-L1 in
plasma cells is different. Its expression level in patients
with MM was higher than those in healthy volunteers and
patients with monoclonal gammopathy of undetermined
significance. In r/r MM, the expression level of PD-L1 is
significantly increased [76]. Similar to that in CHL, the
protein expression level of PD-L1 in myeloma cells is
related to the increase in the PD-L1 copy number. Studies
have shown that targeting PD-1 can improve survival
in myeloma mouse models [77]. Unlike PD-L1, PD-L2 is
not expressed in myeloma cells [78].
In a phase I study, 27
patients with r/r MM were treated
with nivolumab, with a median follow-up time of 65.6
weeks, and 17 patients (63%) had the best remission rate [36]. A
phase II study (NCT02612779) used nivolumab
combined with elotuzumab in the treatment of six patients
with MM and showed a progression-free survival time
of 16.7 months and an objective remission rate of 51.5%,
which were not better than those with monotherapy.
Some clinical trials are also underway, such as that of
nivolumab combined with melphalan for the treatment
of MM (NCT03292263) and nivolumab, carfilzomib,
dexamethasone, and pelareorep combined with r/r MM
(NCT03605719).
A phase I study showed 20 patients (50%) experienced
remission of r/r MM after treatment with pembrolizumab
combined with lenalidomide and low-dose dexamethasone
[79]. At present, this treatment method is not mentioned in
the treatment guidelines for MM, and only a few related
clinical trials have been conducted. Thus, more research
and exploration are needed in the future.
Adverse reactions
Although targeting PD-1/PD-L1 has remarkable efficacy,
it also induces immune-related AEs (irAEs). In principle,
all checkpoint inhibitors can potentially induce irAEs in
any organ, which can occur late after therapy initiation but
possibly also after therapy cessation [80]. As for targeting
PD-1/PD-L1, related irAEs can involve the skin, gastrointestinal
tract, liver, and endocrine system and other organ
systems [81].
irAEs occur for many reasons, which may be related
to the
increase in cytokine production [82]. However, the types
of specific cytokines and their exact roles in the development
of irAEs remain unclear, requiring further research.
irAEs may also be caused by the cross reaction of T cells
and similar antigens on healthy cells [83]. When treating
two patients with fatal fulminant myocarditis and rhabdomyolysis,
Johnson et al. examined the cross-reactivity
theory of the etiology of irAEs and found that one or more
targets of each patient's anti-tumor immune response were
the same as or similar to the antigens normally expressed
in the skeletal muscle and myocardium [84].
Most observed irAEs
were successfully treated with systemic
corticosteroids, but this was not always the case [83].
Hofmann et al. retrospectively reviewed cases with irAEs
due to nivolumab or pembrolizumab treatment and found
that some of these events resolved without treatment, improved
with corticosteroid or other treatments, or were not
resolved [81]. In addition, Horvat et al. recommended that
the threshold for starting systemic corticosteroids should
be low, and if the symptoms do not improve, the threshold
for upgrading the treatment to anti-TNF-α drugs should be
considered within 1 week after treatment with high-dose
corticosteroids [85].
Conclusions
In summary, targeting PD-1 and PD-L1 can block the combination of PD-1 and PD-L1 to enhance the immune response. It has many applications in tumors and is used in clinical immunotherapy for various tumors, with satisfactory therapeutic effects. In addition, with the gradual advancement of research and the increase in technical level, the therapeutic range of targeting PD-1/PD-L1 in tumors has gradually widened, and the remission rate of the disease has gradually increased. However, this treatment method still faces problems such as high drug development costs, instability, potential side effects, and a lack of standardized PD-1 detection procedures. We believe that with the advancement of research and the improvement of the technical level, these issues will be solved or improved. Targeting PD-1/PD-L1 will achieve greater breakthroughs and exert greater value in clinical research and antitumor therapy in the future.
Abbreviations | |
---|---|
AE | adverse events |
ALL | acute lymphocytic leukemia |
AML | acute myeloid leukemia |
APCs | antigen presenting cells |
BICR | blinded independent central review |
BV | brentuximab vedotin |
CLL | chronic lymphocytic leukemia |
CML | chronic myeloid leukemia |
CTLA-4 | Cytotoxic-T-lymphocyte-antigen-4 |
DC | dendritic cel |
DLBCL | diffuse large B-cell lymphoma |
EBV | Epstein-Barr virus |
EHA | The European Hematology Association |
ENKTCL | extranodal NK/T cell lymphoma |
Fc | crystallizable fragment |
FDA | Food and Drug Administration |
FL | follicular lymphoma |
GCB | germinal center B-cell-like |
GVD | gemcitabine, vinorelbine, liposomal doxorubicin |
HL | Hodgkin lymphoma |
IFN γ | interferon γ |
IHC | immunohistochemistry |
IL-2 | interleukin-2 |
irAEs | immune-related adverse events |
IRC | independent review committee |
ITIM | immunoreceptor tyrosine-based inhibitory motif |
ITSM | immunoreceptor tyrosine-based switch motif |
JNK | Janus N-terminal Kinase |
Lag-3 | lymphocyte-activation gene 3 |
LMP1 | latent membrane protein 1 |
LPS | lipopolysaccharide |
MCL | mantle cell lymphoma |
MDS | myelodysplastic syndromes |
MGUS | monoclonal gammopathy of undetermined significance |
MM | multiple myeloma |
MRD | minimal residual disease |
NCCN | National Comprehensive Cancer Network |
NGCB | non-germinal center B-cell-like |
NHL | non-Hodgkin's lymphoma |
NK | natural killer |
NKTCL | NK/T cell lymphoma |
NOS | not otherwise specified |
NSCLC | non-small cell lung cancer |
PCNSL | primary central nervous system lymphoma |
PD-1 | programmed cell death-1 |
PD-L1 | programmed cell death-ligand 2 |
PD-L2 | programmed cell death-ligand 1 |
PD-Ls | programmed cell death-ligands |
P-GEMOX | pegaspargase, gemcitabine, oxaliplatin |
PKC-θ | protein kinase C-θ |
PMBCL | primary mediastinal large B cell lymphoma |
PTCL | peripheral T cell lymphoma |
PTL | primary testicular lymphoma |
r/r HL | relapsed/refractory HL |
R-CHOP | rituximab plus cyclophosphamide, doxorubicin, vincristine, and prednisone |
RGMb | rejection guiding molecule b |
RS | Richter's syndrome |
SHPs | Src homology 2 domain-containing phosphatases |
TCL | T cell lymphoma |
TCR | T-cell receptor |
TFH | follicular helper T cells |
TILs | tumor-infiltrating lymphocytes |
TIM-3 | T cell immunoglobulin and mucin-domain containing-3 |
TLR | Toll-like-receptor |
TNF | tumor necrosis factor |
ZAP70 | zeta-chain-associated protein kinase 70 |
Declarations
Author contributions
Liang Wang designed the study. Wanying Zhao and Yuanzheng Liang collected all the literatures and analyzed the data. Wanying Zhao wrote the paper. All authors revised the paper and approved the publication of this paper.
Availability of data and materials
Not applicable
Financial support and sponsorship
This work was financially supported through grants from the National Natural Science Foundation of China (81873450, 82170181) and the Open Research Fund from Beijing Advanced Innovation Center for Big Data-Based Precision Medicine, Beijing Tongren Hospital, Beihang University & Capital Medical University (grant No. BHTR-KFJJ-202009) to Liang Wang.
Conflicts of interest
Liang Wang is a member of the Editorial Board of Aging Pathobiology and Therapeutics. All authors declare no conflict of interest and were not involved in the journal's review or desicions related to this manuscript.
Ethics approval and consent to participate
Not applicable.
References
1. Couzin-Frankel J. Breakthrough of the year 2013. Cancer immunotherapy. Science, 2013, 342(6165): 1432-1433.
2. Ok C Y, Young K H. Checkpoint inhibitors in hematological malignancies. Journal of Hematology & Oncology, 2017, 10(1): 103.
3. Bachy E, Coiffier B. Anti-PD1 antibody: a new approach to treatment of lymphomas. Lancet Oncology, 2014, 15(1): 7-8.
4. Keir M E, Butte M J, Freeman G J, et al. PD-1 and its ligands in tolerance and immunity. Annual Review of Immunology, 2008, 26(1): 677-704.
5. Zhang X, Schwartz J-C D, Guo X, et al. Structural and Functional Analysis of the Costimulatory Receptor Programmed Death-1. Immunity, 2004, 20(3): 337-347.
6. Ishida Y, Agata Y, Shibahara K, et al. Induced expression of PD-1, a novel member of the immunoglobulin gene superfamily, upon programmed cell death. The EMBO Journal, 1992, 11(11): 3887-3895.
7. Guntermann C, Alexander D R. CTLA-4 Suppresses Proximal TCR Signaling in Resting Human CD4+ T Cells by Inhibiting ZAP-70 Tyr319 Phosphorylation: A Potential Role for Tyrosine Phosphatases. Journal of Immunology, 2002, 168(9): 4420-4429.
8. Okazaki T, Honjo T. The PD-1-PD-L pathway in immunological tolerance. Trends in Immunology, 2006, 27(4): 195-201.
9. Cho H-Y, Choi E-K, Lee S-W, et al. Programmed death-1 receptor negatively regulates LPS-mediated IL-12 production and differentiation of murine macrophage RAW264.7 cells. Immunology Letters, 2009, 127(1): 39-47.
10. Sharpe A H, Wherry E J, Ahmed R, et al. The function of programmed cell death 1 and its ligands in regulating autoimmunity and infection. Nature Immunology, 2007, 8(3): 239-245.
11. Latchman Y, Wood C R, Chernova T, et al. PD-L2 is a second ligand for PD-1 and inhibits T cell activation. Nature Immunology, 2001, 2(3): 261-268.
12. Sugiura D, Maruhashi T, Okazaki I-M, et al. Restriction of PD-1 function by cis -PD-L1/CD80 interactions is required for optimal T cell responses. Science, 2019, 364(6440): 558-566.
13. Patsoukis N, Wang Q, Strauss L, et al. Revisiting the PD-1 pathway. Science Advances, 2020, 6(38): eabd2712.
14. Saunders P A, Hendrycks V R, Lidinsky W A, et al. PDL2: PD-1 involvement in T cell proliferation, cytokine production, and integrin-mediated adhesion. European Journal of Immunology, 2010, 35(12): 3561-3569.
15. Francisco L M, Salinas V H, Brown K E, et al. PD-L1 regulates the development, maintenance, and function of induced regulatory T cells. Journal of Experimental Medicine, 2009, 206(13): 3015-3029.
16. Callahan M K, Postow M A, Wolchok J D. Targeting T Cell Co-receptors for Cancer Therapy. Immunity, 2016, 44(5): 1069-1078.
17. Zhuang Y, Liu C, Liu J, et al. Resistance mechanism of PD-1/PD-L1 blockade in the cancer-immunity cycle. Onco Targets and therapy, 2020, 13: 83-94.
18. Minn A J, Wherry E J. Combination Cancer Therapies with Immune Checkpoint Blockade: Convergence on Interferon Signaling. Cell, 2016, 165(2): 272-275.
19. Twyman-Saint Victor C, Rech A J, Maity A, et al. Radiation and dual checkpoint blockade activate nonredundant immune mechanisms in cancer. Nature, 2015, 520(7547): 373-377.
20. Diggs L P, Hsueh E C. Utility of PD-L1 immunohistochemistry assays for predicting PD-1/PD-L1 inhibitor response. Biomarker Research, 2017, 5(1): 12.
21. Xie M, Huang X, Ye X, et al. Prognostic and clinicopathological significance of PD-1/PD-L1 expression in the tumor microenvironment and neoplastic cells for lymphoma - ScienceDirect. International Immunopharmacology, 2019, 77: 105999.
22. Boussiontis V A. Molecular and Biochemical Aspects of the PD-1 Checkpoint Pathway. New England Journal of Medicine, 2016, 375(18): 1767-1778.
23. Longley J, Johnson P W M. Options for first line therapy of Hodgkin lymphoma. Hematological Oncology, 2019, 37 Suppl 1(Suppl Suppl 1): 82-86.
24. Meti N, Esfahani K, Johnson N A. The Role of Immune Checkpoint Inhibitors in Classical Hodgkin Lymphoma. Cancers (Basel), 2018, 10(6): 204.
25. Bair S M, Strelec L E, Feldman T A, et al. Outcomes and Toxicities of Programmed Death-1 (PD-1) Inhibitors in Hodgkin Lymphoma Patients in the United States: A Real‐World, Multicenter Retrospective Analysis. The Oncologist, 2019, 24(7): 955-962.
26. Md A F H, Phd L C, Md Y N, et al. Consolidation with Nivolumab and Brentuximab Vedotin after Autologous Hematopoietic Cell Transplantation in Patients with High-Risk Hodgkin Lymphoma. Blood, 2020, 136(Supplement 1): 19-20.
27. Chen R W, Zinzani P L, Fanale M A, et al. Pembrolizumab for relapsed/refractory classical Hodgkin lymphoma (R/ R cHL): phase 2 KEYNOTE-087 study. Journal of Clinical Oncology, 2016, 34(15_suppl): 7555.
28. Armand P, Shipp M A, Ribrag V, et al. Programmed death-1 blockade with pembrolizumab in patients with classical Hodgkin lymphoma after brentuximab vedotin failure. Journal of Clinical Oncology, 2016, 34(31): 3733.
29. Moskowitz A J, Shah G, Sch Der H, et al. Phase II Study of Pembrolizumab Plus GVD As Second-Line Therapy for Relapsed or Refractory Classical Hodgkin Lymphoma. Blood, 2020, 136(Supplement 1): 17-18.
30. Su H, Song Y, Jiang W, et al. Sintilimab for relapsed/ refractory classical Hodgkin's lymphoma: Long-term follow-up on the multicenter, single-arm phase II ORIENT- 1 study. American Society of Clinical Oncology, 2020, 38(15_suppl): 8034.
31. Wang C, Liu Y, Dong L, et al. Efficacy of Decitabine plus Anti-PD-1 Camrelizumab in Patients with Hodgkin Lymphoma Who Progressed or Relapsed after PD-1 Blockade Monotherapy. Clinical Cancer Research, 2021, 27(10): 2782-2791.
32. Cheson B D, Bartlett N L, Laplant B, et al. Phase II, multicenter trial of nivolumab (Nivo) and brentuximab vedotin (BV) in patients (Pts) with untreated Hodgkin lymphoma (HL) over the age of 60 years or unable to receive standard ABVD chemotherapy: Results of a study of Academic and Community Cancer Research United (ACCRU) RU051505I. Journal of Clinical Oncology, 2020, 38(15_suppl): 8014.
33. Laurent C, Charmpi K, Ggavelle P, et al. Several immune escape patterns in non-Hodgkin's lymphomas. Oncoimmunology, 2015, 4(8): e1026530.
34. Georgiou K, Chen L, Berglund M, et al. Genetic basis of PD-L1 overexpression in diffuse large B-cell lymphomas. Blood, 2016, 127(24): 3026-3034.
35. Kiyasu J, Miyoshi H, Hirata A, et al. Expression of programmed cell death ligand 1 is associated with poor overall survival in patients with diffuse large B-cell lymphoma. Blood, The Journal of the American Society of Hematology, 2015, 126(19): 2193-2201.
36. Lesokhin A M, Ansell S M, Armand P, et al. Nivolumab in Patients With Relapsed or Refractory Hematologic Malignancy: Preliminary Results of a Phase Ib Study. Journal of Clinical Oncology, 2016, 34(23): 2698-2704.
37. Ansell S, Gutierrez M E, Shipp M A, et al. A phase 1 study of nivolumab in combination with ipilimumab for relapsed or refractory hematologic malignancies (Check- Mate 039). Blood, 2016, 128(22): 183.
38. Smith S D, Till B G, Shadman M S, et al. Pembrolizumab with R-CHOP in previously untreated diffuse large B-cell lymphoma: potential for biomarker driven therapy. British journal of haematology, 2020, 189(6): 1119-1126.
39. Tam C S, Cull G, Opat S, et al. An Update on Safety and Preliminary Efficacy of Highly Specific Bruton Tyrosine Kinase (BTK) Inhibitor Zanubrutinib in Combination with PD-1 Inhibitor Tislelizumab in Patients with Previously Treated B-Cell Lymphoid Malignancies. Blood, 2019, 134 (Supplement_1): 1594.
40. Younes A, Burke J M, Cheson B D, et al. Sharman; Safety and Efficacy of Atezolizumab in Combination with Rituximab Plus CHOP in Previously Untreated Patients with Diffuse Large B-Cell Lymphoma (DLBCL): Updated Analysis of a Phase I/II Study. Blood, 2019, 134(Supplement_ 1): 2874.
41. Chapuy B, Roemer M G M, Stewart C, et al. Targetable genetic features of primary testicular and primary central nervous system lymphomas. Blood, The Journal of the American Society of Hematology, 2016, 127(7): 869-881.
42. Reddy N M, Thieblemont C. Thieblemont C Maintenance therapy following induction chemoimmunotherapy in patients with diffuse large B-cell lymphoma: current perspective. Annals of Oncology, 2017, 28(11): 2680-2690.
43. Nayak L, Iwamoto F, Ferreri A, et al. Chechmate 647: A phase 2, open-label study of nivolumab in relapsed/ refractory primary central nervous system lymphoma or relapsed/refractory primary testicular lymphoma [J]. Hematological Oncology, 2017, 35(S2): 420-421.
44. Westin J R, Fowler N H, Nastoupil L J, et al. A Phase II Trial of Nivolumab and Ibrutinib for Patients with Relapsed or Refractory Central Nervous System Lymphoma. Blood, 2019, 134(Supplement_1): 4086.
45. Chang A, Schlafer D, Flowers C R, et al. Investigational PD-1 inhibitors in HL and NHL and biomarkers for predictors of response and outcome. Expert Opinion on Investigational Drugs, 2018, 27(1): 55-70.
46. Armand P, Rodig S, Melnichenko V, et al. Pembrolizumab in relapsed or refractory primary mediastinal large B-cell lymphoma. Journal of Clinical Oncology, 2019, 37(34): 3291-3299.
47. Zinzani P L, Santoro A, Gritti G, et al. Nivolumab Combined With Brentuximab Vedotin for Relapsed/Refractory Primary Mediastinal Large B-Cell Lymphoma: Efficacy and Safety From the Phase II CheckMate 436 Study. Journal of Clinical Oncology, 2019, 37(33): 3081-3089.
48. Lin N, Song Y, Zhu J. Immune checkpoint inhibitors in malignant lymphoma: Advances and perspectives. Chinese Journal of Cancer Research, 2020, 32(3): 303-318.
49. Garon E B, Rizvi N A, Hui R, et al. Pembrolizumab for the treatment of non-small-cell lung cancer. The New England Journal of Medicine, 2015, 372(21): 2018-2028.
50. Quan L, Chen X, Liu A, et al. PD-1 blockade can restore functions of T-cells in Epstein-Barr virus-positive diffuse large B-cell lymphoma in vitro. PloS one, 2015, 10(9): e0136476.
51. Wahlin B E, Aggarwal M, Montes-Moreno S, et al. A unifying microenvironment model in follicular lymphoma: outcome is predicted by programmed death-1--positive, regulatory, cytotoxic, and helper T cells and macrophages. Clinical Cancer Research, 2010, 16(2): 637-650.
52. Kieser A, Kilger E, Gires O, et al. Epstein-Barr virus latent membrane protein-1 triggers AP-1 activity via the c-Jun N-terminal kinase cascade. The EMBO journal, 1997, 16(21): 6478-6485.
53. Alrawashdh N, Mcbride A, Persky D O, et al. Survival trends in chronic lymphocytic leukemia in the era of oral targeted therapies in the United States: SEER database analyses (1985 to 2017). Journal of Clinical Oncology, 2021, 39(15_suppl): 7524.
54. Westin J R, Chu F, Zhang M, et al. Safety and activity of PD1 blockade by pidilizumab in combination with rituximab in patients with relapsed follicular lymphoma: a single group, open-label, phase 2 trial [J]. Lancet Oncology, 2014, 15(1): 69-77.
55. Karolova J, Radek M, Helman K, et al. PD-1, PD-L1 and PD-L2 Expression in Mantle Cell Lymphoma and Healthy Population. Folia Biologica, 2020, 66(4): 117-122.
56. Vranic S, Ghosh N, Kimbrough J, et al. PD-L1 status in refractory lymphomas. PloS one, 2016, 11(11): e0166266.
57. Han L, Liu F, Li R, et al. Role of programmed death ligands in effective T-cell interactions in extranodal natural killer/T-cell lymphoma. Oncology letters, 2014, 8(4): 1461-1469.
58. Wang H, Wang L, Liu W-J. High post-treatment serum levels of soluble programmed cell death ligand 1 predict early relapse and poor prognosis in extranodal NK/T cell lymphoma patients. Oncotarget, 2016, 7(22): 33035- 33045.
59. Chan T S Y, Li J, Loong F, et al. PD1 blockade with lowdose nivolumab in NK/T cell lymphoma failing L-asparaginase: efficacy and safety. Annals of hematology, 2018, 97(1): 193-196.
60. Kwong Y L, Chan T S Y, Tan D, et al. PD1 blockade with pembrolizumab is highly effective in relapsed or refractory NK/T-cell lymphoma failing l-asparaginase. Blood, 2017, 129(17): 2437-2442.
61. Kim S J, Lim J Q, Laurensia Y, et al. Avelumab for the treatment of relapsed or refractory extranodal NK/T-cell lymphoma: an open-label phase 2 study. Blood, 2020, 136(24): 2754-2763.
62. Cai J, Liu P, Huang H, et al. Combination of anti-PD-1 antibody with P-GEMOX as apotentially effective immunochemotherapy for advancednatural killer/T cell lymphoma. Signal Transduction and Targeted Therapy, 2021, 5(1): 289.
63. Gao Y, Huang H, Wang X, et al. Anti-PD-1 Antibody (Sintilimab) Plus Histone Deacetylase Inhibitor (Chidamide) for the Treatment of Refractory or Relapsed Extranodal Natural Killer/T Cell Lymphoma, Nasal Type (r/r-ENKTL): Preliminary Results from a Prospective, Multicenter, Single-Arm, Phase Ib/II Trial (SCENT). Blood, 2020, 136(Supplement 1): 39-40.
64. Wang J S. Progress and Trend of CAR-T and PD-1 Blockade in the Treatment of Acute Myeloid Leukemia- -Review [J]. Zhongguo shi yan xue ye xue za zhi, 2020, 28(3): 1069-1074.
65. Zhang L, GAJEWSKI T F, KLINE J. PD-1/PD-L1 interactions inhibit antitumor immune responses in a murine acute myeloid leukemia model. Blood, 2009, 114(8): 1545-1552.
66. Md N D, Phd S B, Md G G-M, et al. Defining the immune checkpoint landscape in patients (pts) with acute myeloid leukemia (AML) [J]. Blood, 2016, 128(22): 2900.
67. Kadia T M, Cortes J E, Ghorab A, et al. Nivolumab (Nivo) maintenance (maint) in high-risk (HR) acute myeloid leukemia (AML) patients. Journal of Clinical Oncology, 2018, 36(15_suppl): 7014.
68. Daver N, Garcia-Manero G, Basu S, et al. Efficacy, safety, and biomarkers of response to azacitidine and nivolumab in relapsed/refractory acute myeloid leukemia: a nonrandomized, open-label, phase II study. Cancer discovery, 2019, 9(3): 370-383.
69. Man L M, Morris A L, Keng M. New Therapeutic Strategies in Acute Lymphocytic Leukemia. Current Hematologic Malignancy Reports, 2017, 12(3): 197-206.
70. Christiansson L, S Dderlund S, Svensson E, et al. Loskog ASI Increased Level of Myeloid-Derived Suppressor Cells, Programmed Death Receptor Ligand 1/Programmed Death Receptor 1, and Soluble CD25 in Sokal High Risk Chronic Myeloid Leukemia. PLoS One, 2013, 8(1): e55818.
71. Held S A E, Heine A, Mayer K T, et al. Advances in Immunotherapy of Chronic Myeloid Leukemia CML. Curr Cancer Drug Targets, 2013, 13(7): 768-774.
72. Ramsay A G, Clear A J, Fatah R, et al. Multiple inhibitory ligands induce impaired T-cell immunologic synapse function in chronic lymphocytic leukemia that can be blocked with lenalidomide: establishing a reversible immune evasion mechanism in human cancer. Blood, 2012, 120(7): 1412-1421.
73. Riches J C, Davies J K, Mcclananan F, et al. T cells from CLL patients exhibit features of T-cell exhaustion but retain capacity for cytokine production. Blood, 2013, 121(9): 1612-1621.
74. Wang Y, Sinha S, Dong H, et al. Distinct immune signatures in chronic lymphocytic leukemia (CLL) and Richter's syndrome (RS). Journal of Clinical Oncology, 2018, 36(15_suppl): 7524.
75. Yinjuan M, Yaozhu P. Research update on PD-1/PD-L1 pathway in hematological diseases. Journal of Modern Oncology, 2020, 28(16): 2905-2909.
76. Tamura H, Ishibashi M, Sunakawa-Kii M, et al. PD-L1- PD-1 pathway in the pathophysiology of multiple myeloma. Cancers, 2020, 12(4): 924.
77. Hallett W H D, Jing W, Drobyski W R, et al. Immunosuppressive Effects of Multiple Myeloma Are Overcome by PD-L1 Blockade - ScienceDirect. Biology of Blood and Marrow Transplantation, 2011, 17(8): 1133-1145.
78. G RG N G, Samur M K, Cowens K B, et al. Lenalidomide Enhances Immune Checkpoint Blockade-Induced Immune Response in Multiple Myeloma. Clinical Cancer Research, 2015, 21(20): 4607-4618.
79. Mateos M-V, Orlowski R, Siegel D, et al. Pembrolizumab in combination with lenalidomide and low-dose dexamethasone for relapsed/refractory multiple myeloma: final efficacy and safety analysis. Journal of Clinical Oncology, 2016, 34(suppl 15): 8010a.
80. Zimmer L, Goldinger S M, Hofmann L, et al. Neurological, respiratory, musculoskeletal, cardiac and ocular sideeffects of anti-PD-1 therapy. European Journal of Cancer, 2016, 60: 210-225.
81. Hofmann L, Forschner A, Loquai C, et al. Cutaneous, gastrointestinal, hepatic, endocrine, and renal side-effects of anti-PD-1 therapy. European Journal of Cancer, 2016, 60: 190-209.
82. Oyanagi J, Koh Y, Sato K, et al. Predictive value of serum protein levels in patients with advanced non-small cell lung cancer treated with nivolumab. Lung Cancer, 2019, 132: 107-113.
83. Yoest J M. Clinical features, predictive correlates, and pathophysiology of immune-related adverse events in immune checkpoint inhibitor treatments in cancer: a short review. ImmunoTargets and therapy, 2017, 6: 73- 82.
84. Johnson D B, Balko J M, Compton M L, et al. Fulminant Myocarditis with Combination Immune Checkpoint Blockade. New England Journal of Medicine, 2016, 375(18): 1749-1755.
85. Horvat T Z, Adel N G, Dang T-O, et al. Immune-Related Adverse Events, Need for Systemic Immunosuppression, and Effects on Survival and Time to Treatment Failure in Patients With Melanoma Treated With Ipilimumab at Memorial Sloan Kettering Cancer Center. Journal of Clinical Oncology, 2015, 33(28): 3193-3198.