Open Access | Review
This work is licensed under a Creative
Commons Attribution-ShareAlike 4.0 International License.
Progress on the study of fibroblast growth factors as novel therapeutics in post-stroke cognitive impairment
* Corresponding author: Hong Fan
Mailing address: Department of Geriatrics,Department of Integrated
Traditional Chinese and Western Medicine, Shaoxing People’ s hospital, NO. 568, Zhongxing North Road, Shaoxing
312000, People’ s Republic of China.
E-mail: fhrainbow@163.com
Received: 03 August 2021 / Accepted: 06 September 2021
DOI: 10.31491/APT.2021.09.065
Abstract
Post-stroke cognitive impairment (PSCI) is one of the most common complications after stroke, which severely
affects the daily life abilities and social function of patients. Fibroblast growth factor (FGF), as a
regulator of
homeostasis, participates in the regulation of cell metabolism and hormone secretion, and can increase
cerebral
blood flow and promote nerve repair which improves PSCI. Here, we will conduct a review on the typing,
characteristics, and mechanism of action of FGF to further understand its function and mechanism in PSCI.
Fibroblast growth factor, cognitive impairment, stroke
Keywords
Introduction
Fibroblast growth factors (FGFs) are a class of multifunctional polypeptide molecules, including 23 family members, which are greatly distributed in various parts of the body. FGFs are involved in wound healing, angiogenesis, and neural stem cell proliferation and differentiation, which exert different biological effects under different physiological conditions and influence the whole process of wound healing. Post-stroke cognitive impairment (PSCI) refers to cognitive impairment that appears within six months after stroke events, which varies depending on the different characteristics like the type, volume, number, location, and severity of stroke, including multiple infarctions, cerebral infarction in strategic locations, subcortical ischemia, and cerebral hemorrhages. FGFs increase blood flow to the ischemic penumbra, inhibit vascular endothelial cell apoptosis, thereby mediating early inflammatory response after cell injury, increasing later active repair capacity, promoting angiogenesis and neurological functional recovery, which improve neurological function after stroke. The application of FGFs may open new avenues for PSCI treatment.
The emergence and current treatment of PSCI
Stroke is a common and frequently-occurring disease in
the elderly population of the world, it is the second leading
cause of death worldwide, affecting about 13.7 million
people and leading to about 5.5 million deaths each year
[1]. As societies age and stroke risk factors become generalized,
the incidence and prevalence of stroke continue to
increase and show a rapid increasing trend in low-income
groups [2]. In China, there are over 2 million new stroke
cases every year, among which people aged 20-45 years
rise from 12.9% to 18.6% in the last 30 years [3]. Besides,
the age-standardized attributable mortality rate has decreased
by 36.2% over the same period, which results in
a disability rate as high as 63.1%, leading to the highest
disability rate of all diseases [4]. Around 1/3 of stroke survivors
exist with varying degrees of PSCI, which severely
affects the daily life and social function of patients [5].
The
etiology and pathogenesis of post-stroke
cognitive
impairment are very complex, and are the result of multiple
factors. Currently, it is mainly believed to be related
to the interaction between vascular injury and neurodegeneration.
Neurorestorative progression in stroke is often related to neurogenesis, angiogenesis, and synaptic
plasticity.
The neovascularization at the periphery of stroke
lesions increases cerebral blood flow of ischemic border
zone, which provides oxygen and nutrients for ischemic
region and improves and restores neurological function [6].
Therefore, increasing angiogenesis may become a novel
direction for PSCI treatment.
Currently, the drug treatment of PSCI mainly includes
cholinesterase inhibitors (donepezil), calcium channel antagonists
(nimodipine), and excitatory amino acid receptor
antagonists (memantine, a NDMAR antagonists). Clinical
research has demonstrated that donepezil improves overall
functioning and self-care ability of patients, showing
a role in improving mild and moderate cognitive dysfunction
[45]. Nimodipine has been reported to have the
effects of selectively dilating cerebral blood vessels and
increasing cerebral blood flow, which can improve cognitive
function of PSCI [7]. A previous study revealed that
memantine improves cognitive impairment and mental
status of patients with vascular dementia, but the role of
memantine on PSCI needs further verification [8]. At present,
in addition to drug treatment, the intervention including
cognitive rehabilitation training and transcranial magnetic
stimulation also exhibits certain efficacy for PSCI
treatment. However, cognitive rehabilitation training is
not only affected by patients’ compliance and therapists’
ability level, but also limited by therapeutic equipment
such as transcranial magnetic stimulation, which leads to
the uncertain effect of rehabilitation training. Therefore, it
still lacks a certain effective intervention for PSCI treatment
[9], and the role of FGF in repair related angiogenesis
has been demonstrated in many studies. For example,
targeted FGFR1 and FGFR2 knockout in mouse endothelial
cells inhibits angiogenesis during wound healing, and
it is expected to become a new clinical therapeutics for
PSCI [10].
The definition,types, and mechanism of action of FGFs
Definition and types
FGFs, as a class of polypeptide growth factors, have
multiple biological functions, including cell growth and
differentiation, angiogenesis, embryonic development,
wound healing, and mediating metabolism. FGFs have
conserved core structure β-trilobate fold, thatconsists of
12 strands of β-thin sections arranged around the central
axis into 3 similar lobes, of which 6 strands form an antiparallel
β-barrel fold [11]. According to differences in
sequence homology and development features, the FGF
family can be classified into 6 subfamilies. Most FGFs
are autocrine and paracrine factors, which mediate tissue
and organ generation and metabolism during embryonic
development [12]. FGFs are a group of structurally related
polypeptide mitogen, including 23 different members. Except
for acidic FGFs (aFGF, FGF-1) and basic FGF (bFGF,
FGF-2), FGF family also includes int-2 (FGF-3), protooncogene
products (FGF-4, FGF-5, FGF-6), keratinocyte growth factor (KGF, FGF-7), and androgen-induced
growth factors (AIGF, FGF-8, GAF, FGF-9, FGF-10).
Members of FGF family promote fibroblast proliferation
and activation by stimulating collagen accumulation
and endothelial cell differentiation [13]. Therefore, FGFs
stimulate angiogenesis, thereby playing a critical role during
cell repair.
Fibroblast growth factor (FGF) and its receptor (FGFR)
are conserved transmembrane growth factor receptors that
are widely distributed in all vertebrate tissues, and FGFs
and FGFRs share signal homology between their respective
groups as well as with other signaling molecules. In
humans, there are five known FGFRs, known as FGFR1
– FGFR4 and FGFRL1 (also known as FGFR5). FGFR1
– FGFR4 is a typical growth factor receptor tyrosine
kinase with an extracellular immunoglobulin (Ig) -like
domain and an intracellular tyrosine kinase domain, while
FGFRL1 lacks an intracellular kinase domain. Different
members of the FGF family bind to four different FGF receptors
(FGFR1-FGFR4) and function in complementary
ways. FGFR closely resembles other signaling receptors,
including vascular endothelial growth factor receptor
(VEGFR), platelet-derived growth factor receptor (PDGFR),
and other receptor tyrosine kinases. However, there
are important differences between individual signaling
molecules that can precisely control the entire process, including
development, cell survival, differentiation, motility,
angiogenesis, and carcinogenesis [14].
Characteristics
A majority of FGFs have a very broad mitogenic spectrum, which have multifarious activities and act as neurotrophic and angiogenic factors in vivo. FGFs are widely distributed in vascular endothelium, adrenal cortex, and fibroblast. FGF-5, FGF-8, FGF-9, and FGF-10 subfamilies combine extracellular matrix heparan sulfate and drive autocrine/ paracrine mitogenic signaling axis to promote cell proliferation, while FGF-19, FGF-21, and FGF-23 do not directly promote proliferation [15]. The different members of FGF family bind to four distinct FGF receptors (FGFR1, 2, 3, 4) and act in a complementary manner, thereby producing multiple biological phenomena, such as development, differentiation, migration, survival, cell division and proliferation, and wound healing [16]. The majority of FGFs, as autocrine and paracrine factors, mediate tissue and organ generation during embryonic development, interact with heparin or heparin sulfate, enhance the activity and generation of brain cells and peripheral nerve cells, further exerting a role on PSCI repair [12]. FGFs maintains the activity and function of damaged cells surrounding lesions by inducing chemical activity and promoting angiogenesis, thereby reducing cell necrosis and improving ischemic/hypoxic injury states. FGFs further promote cell survival, which provide conditions for the maintenance and rehabilitation of injured tissue function and are effective in the rehabilitation of consciousness, language, and muscle strength. Thus, FGFs may play a role in PSCI and improve its prognosis [14].
The effects of FGFs on PSCI FGF-1 (aFGF)
Fibroblast growth factor-1 (FGF-1) is a typical member of
the FGF family, which participates in various biological
processes, including cell growth, proliferation, survival,
and development. FGF-1 stimulates cellular signaling
pathways like PKC/PLCγ, PI3K/Akt, and Ras/MAPK by
combining and activating specific receptor FGFR [17].
Uchida et al. [18] found that the continuous expression of
Fgf1b gene in the CA regions of the hippocampus after
learning was related to the increase of memory strength,
and Fgf1b was expressed specifically in the CA regions of
the hippocampus, indicating that the CA regions may be
related to the establishment of memory strength. In addition,
the levels of FGF-1 in the hippocampus were reduced
by human intervention after intense memory training.
The instantaneous memory of experiment subjects was
not affected, but the long-term memory was decreased.
Therefore, FGF-1 may be a necessary condition for the
transformation of memory from instantaneous plasticity
to strong enduring plasticity. Chang et al. [19] compared
97 cases of Alzheimer’ s disease (AD) patients carrying
genomic variants of different FGF-1 and compared the
effects of different genotypes on episodic memory impairment
and hippocampus atrophy (HA). Next, they found
that the clinicopathological relationship of AD exists genotype
effects, and FGF-1 plays a role in episodic memory
(EM) deficits. FGF-1 promotes neurodegenerative related
neurons and synaptic dysfunction and enhances the invasion
capacity of fibroblasts by promoting neuron survival,
inhibiting neurotoxicity, and preventing nerve injury, further
transforming to functional neurons and promoting the
recovery of PSCI.
Hamed et al. [20] first explored the effects of
acute intravenous
administration of adipose-derived mesenchymal
stem cells transfected with FGF-1 into mice with transient
experimental ischemic stroke. The neurological functional
recovery in cerebral ischemic regions, infarct size, and
the expression of FGF-1 protein were determined, and the
results found that FGF-1 treatment significantly improves
neurological function, increases the intensity of FGF-1
protein in perinfarct area, while the infarct size and the
apoptotic index are markedly decreased. All in all, acute
intravenous administration of FGF-1 may become a new
way for the treatment of PSCI in the future. Also, a genetic
study revealed that FGF-1 may be involved in AD
due to the obvious association of single nucleotide polymorphisms
in FGF-1 gene, and the number of FGF-1-immunolabeled
neurons decreases in AD patients, indicating
that FGF-1 deficiency may be related to the pathophysiology
of AD [21]. The increase of FGF-1 levels exerts a
significant effect on glial cells and capillary proliferation,
neurite outgrowth, and synapse formation. Therefore,
FGF-1 may promote neuron and capillary repair, and improve
its prognosis.
FGF-2 (bFGF)
Basic fibroblast growth factor (bFGF), as a member of FGF superfamily, plays a critical role in angiogenesis and neuron survival. FGF-2 may promote neural stem cells in the subgranular zone of hippocampal dentate gyrus and the subventricular zone of the lateral ventricle to generate new neurons. The function of FGF-2 is integrated into mature neural networks to promote the growth, differentiation, maintenance, and survival of new neurons and synapses, or to stimulate the growth of new blood vessels needed for brain tissue vascularization. New neurons may contribute towards stress response and adaptation, learning, and memory due to the sensitivity and high plasticity to environmental stimuli. The abnormal neurogenesis and the impairment of growth factor function, especially hippocampus, are linked to the appearance of neurologic emotion and neurocognitive symptoms [22]. Lin et al. [23] explored the protective mechanism of FGF-2 on BBB by measuring the permeability of endothelial cell monolayers induced by oxygen and glucose deprivation/reoxygenation (OGD/R) and found that FGF-2 significantly reduces the permeability of primary human brain microvascular endothelial cell (HBMEC) monolayers induced by OGD/ R and decreases the transendothelial electrical resistance. These data demonstrated that recombinant FGF-2 has a protective effect on BBB disruption in traumatic brain injury mice, suggesting that FGF-2 is a potential therapy for treating BBB damage in nervous system diseases. Seo et al. [24] investigated angiogenesis in ischemia/hypoxiainduced mouse brain damage model and measured FGF- 2 expression in frontal cortex, striatum, and cerebellum. They further found that the levels of FGF-2 were significantly upregulated in the frontal cortex after 8 weeks of treatment, supporting that FGF-2 promotes endogenous angiogenesis and neurobehavioral functioning, which may be applied to the treatment of PSCI or other nervous system diseases.
FGF-10
FGF-10, a member of the FGF family, is a multifunctional growth factor, which has been extensively studied in the repair and wound healing after cerebral ischemic injury [25]. Li et al. [26] found that injection of FGF-10 into the lateral ventricle of middle cerebral artery occlusion (MCAO) mice significantly reduces infarct area (from 32% to 20%) and improves the neurological deficits, and the mechanism is related to decreased apoptosis. Also, FGF-10 improves cerebral blood flow in ischemic region and may have reparative effects on PSCI, thus being applied in the treatment of PSCI. Fang et al. [27] found obvious cerebral hemisphere edema in the ligation side 24 h after ligation of common carotid artery in neonatal mice to stimulate hypoxic/ischemic encephalopathy (HI). Also, they further demonstrated that intraperitoneal injection of FGF-10 markedly reduced brain edema, proving that FGF-10 treatment can significantly reduce brain damage after HI. Chen et al. [28] simulated spinal cord injury by resection of partial spinal cord in rats and performed immunofluorescence staining on the spinal cord tissues near the lesions. Further, they found that central gray matter and peripheral white matter are visibly damaged and anterior horn motor neuron loss is obvious in the traumatic spinal cord injury (SCI) group. While the necrotic cavity around the injured site was reduced and anterior horn motor neuron loss was decreased in the FGF-10 treatment group. These evidences indicate that FGF-10 has a protective effect on severe injury after SCI, suggesting that FGF-10 is a potential therapy for the treatment of central nervous system damage and has a certain application value for PSCI.
FGF-13
FGF-13 is a member of FGF homologous factor (FHF) subfamily, which is widely expressed in the central nervous system. FGF-13 is located on X chromosomes, and FGF-13 deficiency results in Forssman-Lehmann syndrome. Therefore, FGF-13 is considered as a related gene of intellectual development. During brain development, FGF-13 mediates neuronal cell growth and development through stabilizing tubulin [29]. Poulian et al. [30] found delayed neuronal migration and severe mental retardation in FGF-13 knockdown mice, indicating that FGF-13 plays an essential role in establishing neural circuits in the cerebral cortex and achieving cognitive function. Yuan et al. [31] demonstrated that FGF-13 is essential for excitation/ inhibition balance of synapse in FGF-13 deficiency and replication mouse model. FGF-13-related neural circuits control the emotion and cognitive behavior of autism and mental retardation, which exerts different effects on mediating emotion and cognitive behavior. Therefore, the dysregulated FGF-13 expression may have a broad effect on neuronal and synaptic homeostasis and nervous system functions, which serves a major role in PSCI. Voltage-gated sodium channels (VGSCs) in the axon initial segment (AIS) is critical in initiating action potentials effectively. Pablo et al. [32] employed gene knockout strategies and revealed that FGF-13 binds to VGSC in hippocampal neurons to restrict its expression in somatic cell surface. Besides, experimental data indicated that FGF-13 and FGF-14 acts in concert to regulate VGSC polarization, further supports effective action potentials initiation, regulates outbreaks in the action potentials of neural cells, and stimulates the incidences of cognitive function, showing a certain positive influence on improving PSCI.
FGF-21
FGF-21 is a member of the endocrine FGF family [33],
which has powerful polymetabolic effects [34]. Accumulating
evidence has indicated that FGF-21 is involved in
tissue injury and repair under various pathological conditions
[35]. Jiang et al. [36]
found that metabolic disorders
improved in stroke mice after 14 days of daily treatment
with recombinant human fibroblast growth factor 21
(RFGF-21) in a diabetic mouse model.Importantly, there
was a significant reduction in white matter damage and
neurological deficits between 6 h and 14 d of treatment
with rFGF-21, indicating that FGF-21 has a protective
effect on nervous system and may improve the prognosis
of PSCI. Wang et al. [37] induced a mouse model with a high-fat diet
and found that subcutaneous injection of
rFGF-21 significantly increases FGFR1 concentration in
cerebrospinal fluid and enhances the levels of phosphorylated
FGFR in brain tissues, suggesting that rFGF-21 can
cross the blood-brain barrier (BBB) and functionally activate
the FGF21-FGFR1 signaling pathway in the brain.
Further, rFGF-21 can systematically inhibit inflammation,
slow down the occurrence of brain edema, have a long
effective window period inthe injured site, and quickly
activate the intracranial pathway, which is a molecular
target of novel disease course adjustment strategies and
has certain application value for PSCI. Yang et al. [38] in
a study of ischemia/reperfusion rat model, revealed that
FGF-21 decreases oxidative stress, protects and repairs injured
neurons after ischemia/reperfusion, improves brain
damage by activating ERK1/2 and PI3K/Akt signaling
pathways, which may have reparative effects on PSCI.
The related results are detailed in Figure 1 and Figure
2.
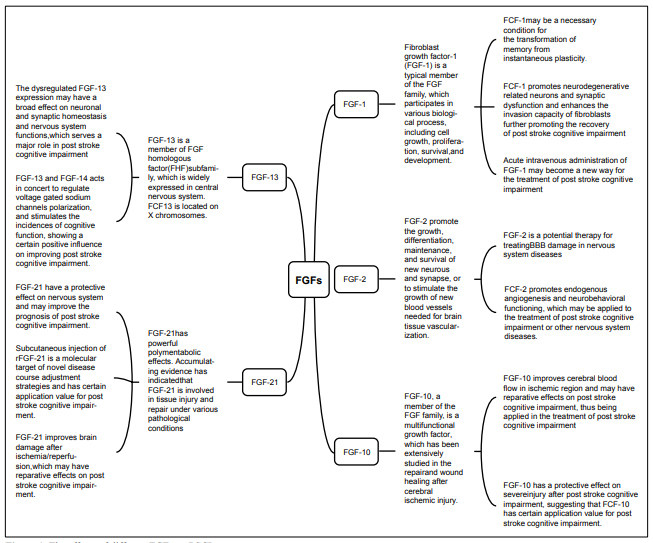
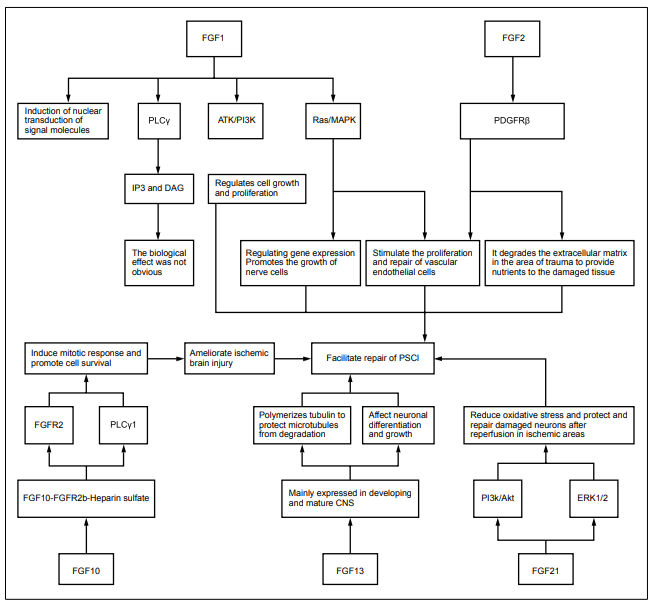
The mechanism of action of FGFs on improving PSCI
Brain function and plasticity rely on the establishment of neural circuits during development. Neuronal morphogenesis, cell migration, and synapse formation defects in the cerebral cortex cause mental retardation, cognitive deficit, and other symptoms [39]. Neural stem cells are mainly distributed in the subventricular and the dentate gyrus, and which actively proliferate in the subventricular and the dentate gyrus after traumatic brain injury, suggesting that the brain has the potential for the repair of injured cells and alleviates brain damage by promoting neural stem cell proliferation and division in these regions. The main method is to promote neural stem cell proliferation through mitogenic factors [9]. FGF is a type of effective stem cell mitogen involved in multiple cellular processes like stem cell differentiation, proliferation, anti-apoptosis, and angiogenesis. Secreted FGFs (FGF1-10 and FGF15- 23) contain an N terminal signal peptide that promote the formation of axon branching by enhancing microtubulessevering. Prior study has demonstrated that injection of FGF into damaged areas induces the proliferation and division of injured neural stem cells [46]. Theinjection of FGF into the animals obviously improve cognitive function, suggesting that FGF may promote PSCI recovery [40]. BBB disruption after stroke causes intracranial inflammatory response, brain edema, and central neurological impairment, thereby inducing PSCI. Huang et al. [41] found that FGF protects the integrity of BBB, controls the degree of brain edema, and repairs damaged nerves, thus reducing the occurrence of PSCI and improving its prognosis. It has been reported that supplementation with exogenous FGF-1, FGF-2 protects neuronal cells, increases transmitter release, thereby effectively alleviating the development of PSCI [42]. FGF-9 suppresses the apoptosis of striatal cells and ameliorates PSCI development through regulating ERKs signaling pathway [43]. Gimeno et al. [44] found that FGFs are expressed in various regions including the thalamus and the interthalamic boundary zone at the early neural development of mice and expressed in cochlea and midbrain at later stages. Further, they revealed that FGFs regulate the proliferation and survival of dorsal cell populations via mediating the mitogen responsiveness of dorsal neuronal precursor, suggesting that FGFs may also have the properties of morphogenesis in brain. Until today, there are plenty of studies supporting that FGF-related factors can maintain and repair injured nerve cells after stroke, and delay the progression of stroke and improve its prognosis, thereby preventing/improving PSCI [1].
Conclusions and perspectives
The main cause of PSCI is impaired nervous system function resulted from the ischemia and hypoxia of brain cells. Until present, only intravenous thrombolysis is relatively effective for post-stroke rehabilitation but with a narrow time window, which limits its application. Currently, there still lacks effective measures to prevent and treat PSCI clinically, so it is of great significance to explore new drugs to ameliorate neuronal cell damage after stroke and to reduce the incidence of PSCI. FGFs control cells and hormones that are involved in metabolism. Also, FGFs have multiple targets of pharmacological effects in the treatment of ischemic stroke, including directly promoting neuronal survival, enhancing angiogenesis, preventing BBB from damage, and mediating microglia regulation, which can be utilized as effective drugs for stroke treatment and PSCI prevention. Although FGFs have not been applied in clinical practice, lots of animal experiments have proved that FGFs exert a role in nervous system rehabilitation, vascular repair, and synapses establishment. Consequently, FGFs are expected to be novel therapeutics for the prevention and treatment of PSCI in the future and are able to elevate the life quality of patients after stroke.
Declarations
Acknowledgments
I would like to gratefully acknowledge the contributions of my friend Jiacheng Wu.
Conflict of interest
The authors declared that there is no conflict of interest.
Authors’ contributions
The author contributed solely to the article.
Financial support and sponsorship
This work was funded by the Zhejiang Basic Public Welfare Research Project(Grant no. LGF20H170005) and Traditional Chinese Medicine Scientific Research Fund Project of Zhejiang Province (Grant no. 2020ZB270).
Ethical approval and consent to participate
Not applicable.
Consent for publication
Not applicable.
References
1. Wolters FJ, Ikram MA. Epidemiology of Vascular Dementia. Arteriosclerosis, Thrombosis, and Vascular Biology, 2019, 39(8): 1542-1549.
2. Haque R, Kabir K, Hossain M, et al. Knowledge of Stroke and its Risk Factors among Stroke Survivors: A Hospital Based Study. Mymensingh medical journal, 2021, 30(2): 376-386.
3. Kuriakose D, Xiao Z. Pathophysiology and Treatment of Stroke: Present Status and Future Perspectives. International Journal Of Molecular Sciences, 2020, 21(20): 7609.
4. Crichton SL, Bray BD, McKevitt C, et al. Patient outcomes up to 15 years after stroke: survival, disability, quality of life, cognition and mental health. Journal of Neurology, Neurosurgery and Psychiatry, 2016, 87(10): 1091-1098.
5. Fride Y, Adamit T, Maeir A, et al. What are the correlates of cognition and participation to return to work after first ever mild stroke? Topics In Stroke Rehabilitation, 2015, 22(5): 317-325.
6. Dordoe C, Chen K, Huang W, et al. Roles of Fibroblast Growth Factors and Their Therapeutic Potential in Treatment of Ischemic Stroke. Frontiers in Pharmacolog, 2021, 12: 671131.
7. J MD, Zito PM. Nimodipine. In: StatPearls. Edn. Treasure Island (FL): StatPearls Publishing Copyright © 2021, StatPearls Publishing LLC, 2021.
8. Swerdlow NR, Bhakta SG, Talledo J, et al. Memantine effects on auditory discrimination and training in schizophrenia patients. Neuropsychopharmacology, 2020, 45(13): 2180-2188.
9. Mijajlović MD, Pavlović A, Brainin M, et al. Post-stroke dementia – a comprehensive review. Bmc Medicine, 2017, 15(1): 11.
10. House S L, Castro A M, Lupu T S, et al. Endothelial fibroblast growth factor receptor signaling is required for vascular remodeling following cardiac ischemia-reperfusion injury. American Journal of Physiology. Heart and Circulatory Physiology, 2016, 310(5): H559-H571.
11. Cho CH, Song KS, Kim BS, et al. Biological Aspect of Pathophysiology for Frozen Shoulder. Biomed Research International, 2018, 2018: 7274517.
12. Kondo Y, Komaba H, Fukagawa M. Endocrine fibroblast growth factors as potential biomarkers for chronic kidney disease. Expert Review Of Molecular Diagnostics, 2020, 20(7): 715-724.
13. de Araújo R, Lôbo M, Trindade K, et al. Fibroblast Growth Factors: A Controlling Mechanism of Skin Aging. Skin pharmacology and physiology, 2019, 32(5): 275-282.
14. Xie Y, Su N, Yang J, et al. FGF/FGFR signaling in health and disease. Signal Transduction and Targeted Therapy, 2020, 5(1):181.
15. Maddaluno L, Urwyler C, Werner S. Fibroblast growth factors: key players in regeneration and tissue repair. Development, 2017, 144(22): 4047-4060.
16. Presta M, Chiodelli P, Giacomini A, et al. Fibroblast growth factors (FGFs) in cancer: FGF traps as a new therapeutic approach. Pharmacology & Therapeutics, 2017, 179: 171-187.
17. Sluzalska KD, Slawski J, Sochacka M, et al. Intracellular partners of fibroblast growth factors 1 and 2 – implications for functions. Cytokine and Growth Factor Reviews, 2021, 57: 93-111.
18. Uchida S, Teubner BJW, Hevi C, et al. CRTC1 Nuclear Translocation Following Learning Modulates Memory Strength via Exchange of Chromatin Remodeling Complexes on the Fgf1 Gene. Cell Reports, 2017, 18(2): 352- 366.
19. Chang YT, Kazui H, Ikeda M, et al. Genetic Interaction of APOE and FGF1 is Associated with Memory Impairment and Hippocampal Atrophy in Alzheimer’s Disease. Aging And Disease, 2019, 10(3): 510-519.
20. Ghazavi H, Hoseini SJ, Ebrahimzadeh-Bideskan A, et al. Fibroblast Growth Factor Type 1 (FGF1)-Overexpressed Adipose-Derived Mesenchaymal Stem Cells (ADMSC( FGF1)) Induce Neuroprotection and Functional Recovery in a Rat Stroke Model. Stem Cell Reviews And Reports, 2017, 13(5): 670-685.
21. Uchida S, Shumyatsky GP. Epigenetic regulation of Fgf1 transcription by CRTC1 and memory enhancement. Brain Research Bulletin, 2018, 141: 3-12.
22. Audet MC, Anisman H. Interplay between pro-inflammatory cytokines and growth factors in depressive illnesses. Frontiers In Cellular Neuroscience, 2013, 7: 68.
23. Lin L, Wang Q, Qian K, et al. bFGF Protects Against Oxygen Glucose Deprivation/Reoxygenation-Induced Endothelial Monolayer Permeability via S1PR1-Dependent Mechanisms. Molecular Neurobiology, 2018, 55(4): 3131-3142.
24. Seo JH, Yu JH, Suh H, et al. Fibroblast growth factor-2 induced by enriched environment enhances angiogenesis and motor function in chronic hypoxic-ischemic brain injury. PloS One, 2013, 8(9): e74405.
25. Tan X, Zhu H, Tao Q, et al. FGF10 Protects Against Renal Ischemia/Reperfusion Injury by Regulating Autophagy and Inflammatory Signaling. Frontiers In Genetics, 2018, 9: 556.
26. Li YH, Fu HL, Tian ML, et al. Neuron-derived FGF10 ameliorates cerebral ischemia injury via inhibiting NF-κBdependent neuroinflammation and activating PI3K/Akt survival signaling pathway in mice. Scientific Reports, 2016, 6: 19869.
27. Fang M, Jiang S, Zhu J, et al. Protective effects of FGF10 on neurovascular unit in a rat model of neonatal hypoxic- ischemic brain injury. Experimental Neurology, 2020, 332: 113393.
28. Chen J, Wang Z, Zheng Z, et al. Neuron and microglia/ macrophage-derived FGF10 activate neuronal FGFR2/ PI3K/Akt signaling and inhibit microglia/macrophages TLR4/NF-κB-dependent neuroinflammation to improve functional recovery after spinal cord injury. Cell Death & Disease, 2017, 8(10): e3090.
29. Wu QF, Yang L, Li S, et al. Fibroblast growth factor 13 is a microtubule-stabilizing protein regulating neuronal polarization and migration. Cell, 2012, 149(7):1549-1564.
30. Poulain FE, Sobel A. The microtubule network and neuronal morphogenesis: Dynamic and coordinated orchestration through multiple players. Molecular And Cellular Neuroscience, 2010, 43(1): 15-32.
31. Yuan B, Cheng TL, Yang K, et al. Autism-related protein MeCP2 regulates FGF13 expression and emotional behaviors. Journal of Genetics and Genomics, 2017, 44(1): 63-66.
32. Pablo JL, Wang C, Presby MM, et al. Polarized localization of voltage-gated Na+ channels is regulated by concerted FGF13 and FGF14 action. Proceedings of the National Academy of Sciences of the United States of America, 2016, 113(19): e2665-2674.
33. Byun S, Seok S, Kim YC, et al. Fasting-induced FGF21 signaling activates hepatic autophagy and lipid degradation via JMJD3 histone demethylase. Nature Communications, 2020, 11(1): 807.
34. Kharitonenkov A, DiMarchi R. FGF21 Revolutions: Recent Advances Illuminating FGF21 Biology and Medicinal Properties. Trends in endocrinology and metabolism, 2015, 26(11): 608-617.
35. Planavila A, Redondo-Angulo I, Villarroya F. FGF21 and Cardiac Physiopathology. Frontiers in Endocrinology, 2015, 6: 133.
36. Jiang Y, Liu N, Wang Q, et al. Endocrine Regulator rFGF21 (Recombinant Human Fibroblast Growth Factor 21) Improves Neurological Outcomes Following Focal Ischemic Stroke of Type 2 Diabetes Mellitus Male Mice. Stroke, 2018, 49(12): 3039-3049.
37. Wang Q, Yuan J, Yu Z, et al. FGF21 Attenuates High-Fat Diet-Induced Cognitive Impairment via Metabolic Regulation and Anti-inflammation of Obese Mice. Molecular Neurobiology, 2018, 55(6): 4702-4717.
38. Yang X, Hui Q, Yu B, et al. Design and Evaluation of Lyophilized Fibroblast Growth Factor 21 and Its Protection against Ischemia Cerebral Injury. Bioconjugate Chemistry, 2018, 29(2): 287-295.
39. Goulay R, Mena Romo L, Hol EM, et al. From Stroke to Dementia: a Comprehensive Review Exposing Tight Interactions Between Stroke and Amyloid-β Formation. ranslational Stroke Research, 2020, 11(4): 601-614.
40. Qiang L, Yu W, Liu M, et al. Basic fibroblast growth factor elicits formation of interstitial axonal branches via enhanced severing of microtubules. Molecular Biology Of The Cell, 2010, 21(2): 334-344.
41. Huang B, Krafft PR, Ma Q, et al. Fibroblast growth factors preserve blood-brain barrier integrity through RhoA inhibition after intracerebral hemorrhage in mice. Neurobiology Of Disease, 2012, 46(1): 204-214.
42. Zhu G, Chen G, Shi L, et al. PEGylated rhFGF-2 conveys long-term neuroprotection and improves neuronal function in a rat model of Parkinson’s disease. Molecular Neurobiology, 2015, 51(1): 32-42.
43. Yusuf IO, Cheng PH, Chen HM, et al. Fibroblast Growth Factor 9 Suppresses Striatal Cell Death Dominantly Through ERK Signaling in Huntington’s Disease. Cellular Physiology And Biochemistry, 2018, 48(2): 605-617.
44. Gimeno L, Hashemi R, Brûlet P, et al. Analysis of Fgf15 expression pattern in the mouse neural tube. Brain Research Bulletin, 2002, 57(3-4): 297-299.
45. Kumar A, Gupta V, Sharma S. Donepezil. 2021.
46. Klimaschewski L, Claus P. Fibroblast Growth Factor Signalling in the Diseased Nervous System. Molecular Neurobiology, 2021,58(8): 3884-3902.